Use of Visual Evoked-Potential Studies and EEG Data to Classify Aggressive, Explosive Behavior of Youths
Abstract
OBJECTIVE: Data from electroencephalograms (EEGs), including data from visual evoked-potential studies, were analyzed to assess their association with a specific type of explosive behavior in children and adolescents. METHODS: Data for 326 children and adolescents treated in a psychiatric clinic were examined. Eighty-two percent exhibited behavior consistent with intermittent explosive disorder, although diagnosis was not an inclusion criterion for the study. The presence of explosive behaviors was indicated by reports from the legal system, schools, parents, health care workers, and psychiatric intake interviews. A quantitative EEG and a series of pattern-reversal evoked-potential studies were administered to each patient. In these studies, children are shown a rapidly reversing checkerboard pattern or rapid flashes of light, and their brain waves (evoked potentials) are measured. RESULTS: Logistic regression indicated that patients who exhibited explosive behaviors were significantly more likely to produce high-amplitude P100 wave forms in the evoked-potential studies than patients who did not exhibit explosive behaviors. Forty-six percent of those with explosive behaviors met the clinically defined electrophysiological criteria for the high-amplitude P100 wave forms. CONCLUSIONS: The use of visual evoked-potential studies helped us classify a large subset of youths who exhibited out-of-control explosive behaviors. The findings suggest that a subgroup of individuals exhibiting explosive behaviors may have a predisposition for violent or explosive behavior that is an innate characteristic of their central nervous system. An understanding of the etiology of explosive behaviors permits the use of more appropriate intervention and treatment strategies.
With each violent outburst by an adolescent in our society, early identification of children who exhibit explosive, aggressive, and uncontrollable outbursts becomes more critical. This difficult population was identified more than 30 years ago by Stevens and colleagues (1).
The deterioration of family structure and the noticeable moral decline in society (2) have only increased the need to accurately identify individuals with explosive and aggressive tendencies and to develop methods to help them achieve success in the world around them. In a study by Cadoret and associates (3), a significant amount of the variability in the aggressivity of adopted children, as well as in conduct disorder and adult antisocial behavior, was accounted for by environmental effects and genetic-environmental interactions. Empirical evidence of these interactions is important to both basic science and applied social sciences for intervention and prevention. As Elliott (4) noted, "As with all behavior, aggression is the result of interactions between the brain (including psychological experience) and environmental challenges—a biopsychosocial phenomenon."
Although environmental factors are important, a review of the literature reveals that many aggressive behaviors have a biological basis, and when such behaviors are not identified and addressed appropriately, positive treatment outcomes are extremely difficult to obtain (5). Matthews and his colleagues (5) have labeled some patients "neurobehavioral" because they believe that underlying abnormalities in brain function are the primary cause of their explosive behavioral outbursts.
Studies evaluating the electrophysiological correlates of aggressive, explosive, and assaultive behaviors have relied primarily on standard electroencephalogram (EEG) data (6,7,8,9,10,11). In two previous studies, we evaluated the relationship between pattern reversal, visual evoked potentials, and explosive behaviors. An evoked potential is a regular pattern of electrical activity recorded from neural tissue that is evoked by a controlled stimulus. In pattern-reversal visual evoked-potential studies, a subject is shown a rapidly alternating pattern, and the subject's EEG data are examined for amplitude and latency of the wave forms, the symmetry of the responses, and any abnormal activity. Such abnormalities often indicate difficulties with behavior and learning. The etiology of explosive behaviors may be different in children who exhibit such abnormalities.
In our first study of 278 children and adolescents, 71 (26 percent) exhibited explosive behaviors, and 237 (85 percent) exhibited at least one type of brain electrical abnormality (12). The other study compared six explosive individuals (four males and two females) who were matched by age, sex, and handedness with six children who did not have a psychiatric diagnosis (13). The mean±SD age of the children was 13.5±2.06 years. In the pattern-reversal evoked-potential studies, the children with explosive behaviors were found to have significantly higher N75 and P100 wave forms than the controls.
This study investigated whether a relationship exists between quantitative EEG data, visual evoked potentials, and explosive behavior of children and adolescents referred to our clinic. During the exploratory studies (12,13), one variable in the visual evoked-potential series—the P100 wave form—consistently showed a significant relationship with explosive behavior. Therefore, in the study reported here we hypothesized that the amplitude of the P100 wave form in the pattern-reversal visual evoked-potential studies would be significantly related to explosive, aggressive, and out-of-control behaviors.
The data we used are a subset of an extensive database gathered over two years involving electrophysiological parameters of various psychiatric behaviors. It should be emphasized that our research deals with behaviors and not diagnostic categories, because explosive behaviors cut across a variety of diagnoses. The results of this study provide possible evidence of a specific class of explosive disorders and may lead to more effective treatment based on neurophysiology and scientific principles.
Methods
An evaluation of electrophysiological, behavioral, and medication data was conducted for all children through 18 years of age who successfully completed a standard protocol of computerized electroencephalographic and evoked-potential evaluations during 1995 and 1996. Before coming for evaluation, many of these patients had been placed in one or more residential treatment facilities and had been seen by several therapists and doctors, yet their uncontrollable behaviors continued.
Of the 454 children and adolescents evaluated, complete data were available for 326—105 females (32 percent) and 221 males (68 percent)—who were included in this study. Children excluded from the study were those who had only a sleep-deprived EEG—that is, those for whom evaluation of evoked potentials was not completed or those who did not correctly count the number of target stimuli given during the auditory-event-related potential studies. We did not consider the amplitude of wave forms or any specific behavioral characteristics for the children not included in the study.
The mean±SD age of the 326 children was 13.27±2.92 years; the mean age of those with explosive behaviors was 13.03±2.95 years, and the age of those without explosive behaviors was 14.32±2.52. Explosive behavior was defined as that with apparent limbic system involvement. Typically it occurs illogically, in situations that would not be expected to prompt an outburst. Such children control themselves in many situations in which a strong emotional response would be understandable; however, an inconsequential situation causes an "explosion" of emotions. The outburst lasts at least 20 minutes and sometimes goes on for a day or more. When the children are approached, the behavior is escalated, and the length of the outburst is extended. Usually they are apologetic after the outburst, and they sometimes deny remembering what occurred.
In our study, an individual was considered to be explosive if any mention of explosive rage, out-of-control anger, out-of-control aggression, verbal or physical attacks on another individual, intermittent explosive disorder, or episodic dyscontrol syndrome was recorded in the clinical file. A total of 267 of the 326 children (82 percent) met these criteria. In our 1991 exploratory study, only 26 percent of the sample exhibited explosive behaviors. The difference reflects an increase in the proportion of explosive individuals among our clinical population because of our clinic's growing reputation for working with explosive, out-of-control individuals.
To account for any effect medication might have had on the electrophysiological data, a dichotomous variable, medications, was created, which indicated whether or not an individual was taking medication at the time of testing.
Informed consent was given by the participants or their parents or guardians after we reviewed the procedure and answered their questions.
Electrophysiological procedure
Each patient underwent a series of evoked-potential studies and a quantitative EEG in accordance with guidelines of the American Electroencephalographic Society (14,15). We used the Brain Atlas III, a product of the Bio-Logic Corporation in Chicago. Electrode placements were in accordance with the international 10-20 system, using an Electro-Cap, with 16 active electrodes. A monopolar montage with forehead ground with linked-ear reference was used. Electrode impedance was maintained at less than 2 kohms, and the impedance between homologous sites was maintained within 1 kohm. The gain was set at 30,000, the low-pass filter at 100 Hz, the high-pass filter at 1 Hz, and the 60-Hz notch filter was set to the "in" position.
Patients were comfortably seated in a padded reclining chair in a small, sound-attenuated room. A channel-by-channel calibration was performed before and after each recording session.
The electrophysiological test series consisted of four visual evoked-potential studies, including pattern reversal (both eyes, left eye, and right eye) and flash (both eyes); three auditory-event-related potential studies (the odd-ball paradigm at three different speeds); two brainstem auditory evoked potentials; and 20 minutes (after two minutes of hyperventilation) of computerized electroencephalography. Digital EEG data were evaluated, and artifact-free data were used to create eyes-open and eyes-closed (resting) fast Fourier transformed files. We analyzed data on visual evoked potentials and fast Fourier transformed files.
Visual evoked potentials
Data from the pattern-reversal visual evoked-potential evaluation were recorded for each individual in accordance with guidelines of the American Electroencephalographic Society (14,15). The checkerboard pattern-reversal paradigm used 19 millimeter, black-and-white alternating squares displayed on a model TC1115 RCA monitor positioned at eye level, 76 centimeters in front of the patient, subtending a visual angle of 23 degrees. The pattern reversed every .59 seconds for a total of 1.7 stimuli per second. A 256 ms epoch was used with a 5 ms prestimulus time. The flash paradigm used a 512 ms epoch with 10 ms of prestimulus time. The intensity of stimulus from the checkerboard pattern-reversal was 12.69 candelas per square meter (cd/m2) and that of the flash 19.26 cd/m2.
The patient was instructed to visually fixate on a red dot centered on the RCA monitor, not to speak, and to remain relaxed with as little movement as possible throughout the recording time. Artifacts were detected and removed using the Bio-Logic online artifact rejection program. For each patient, 200 artifact-free trials were averaged together to produce the final wave form.
Procedure
Patients' clinical files were reviewed for information about sex, age, medications, head injuries, loss of consciousness, explosive behaviors, narcissism, rumination, symptoms of bipolar disorder, or impulsivity. These clinical variables were evaluated for association with several electrophysiological variables. They included the maximum amplitudes of the pattern-reversal evoked-potential N75 and P100 wave forms at O1 and O2; the flash evoked potential P100 wave form at O1 and O2 and P200 at F3 and F4; the auditory-even-related evoked potential P50 wave form at F3 and F4, the N200 wave form at F3, F4, and CZ; the P300 wave form at CZ and PZ; and the eyes-open FFT data recorded from occipital lobe electrodes, O1 and O2, the frontal lobe electrodes, F3 and F4, two central locations, CZ and PZ, and the temporal lobes, T5 and T6 (16,17,18).
All clinical data except explosivity, sex, age, and medications were excluded from the final analysis because although the concordance decreased slightly when clinical data were removed, we decided that the small decrease in concordance was less important than the inclusion of variables that are subjectively based and that may vary dramatically between professionals.
Logistic regression analysis was used to determine which electrophysiological variables were significantly associated with the presence of explosive behaviors. Stepwise analyses were completed on various combinations of EEG data, data from the evoked-potential evaluations, and clinical information, using a cutoff probability value of .25 to produce the preliminary model.
Interactions between sex, age, medications, and the electrophysiological variables identified in the preliminary model were assessed individually. All interaction effects with p values of .15 or less were added to the preliminary model. We then sequentially assessed variables that had p values in excess of .05, starting with the least significant variables. A confounding variable was defined as one that changed the parameter estimate of a significant variable by 20 percent or more.
To gain a basic understanding of the relationship of evoked potentials to underlying brain electrical activity, correlations were determined between amplitudes of the P100 wave forms in the pattern-reversal evoked-potential studies and the EEG data from the occipital electrodes (O1 and O2).
Results
Stepwise logistic regression analysis of the EEG data alone and the EEG data plus the evoked-potential variables indicated that the concordance percentage increased with the addition of evoked-potential data and that the addition of these data significantly improved the analysis, as shown by the -2LOG L chi square statistic in Table 1.
The final model consisted of five variables, which are shown in Table 1. They were the OP100 wave form—that is, the maximum P100 amplitude at the O1 and O2 electrodes—from the pattern-reversal evoked-potential studies; delta F4—that is, the delta absolute power in the right frontal lobe from the EEG data; sex; age; and medications.
Concordance in the models was determined as follows: All possible pairings of individuals who had explosive behavior and who did not have explosive behavior were created. A pair was defined as concordant if the individual who had explosive behavior was also the individual predicted by the logistic regression model to be the one more likely to have explosive behavior on the basis of physiological predictor variables. A pair was defined as discordant if the model incorrectly predicted that the individual who did not have explosive behavior was more likely to be the individual who had explosive behavior.
Higher amplitudes of the OP100 wave form were significantly associated with explosive behaviors, and the amplitude was somewhat higher for the female explosive individuals. As Table 2 shows, males were more likely than females to exhibit explosive behaviors, and the explosive group was slightly younger. Male explosive individuals were the youngest overall. However, these differences were not statistically significant.
Explosive individuals also had higher delta absolute power at the F4 electrode; the most intense delta F4 activity was found in males. Using a 95 percent reliability level, our clinical criteria require a P100 of 10 microvolts or greater to indicate a predisposition to explosive behavior (Figure 1).
In both regression models shown in Table 1, the absolute power of delta F4 was significantly higher in the explosive group than in the nonexplosive group. In the model that included the EEG data plus the evoked-potential variables, the OP100 was significantly correlated with explosive behavior. The medication variable was not significantly associated with explosive behavior in either model. A further set of analyses was performed in which the subjects taking medication at the time of testing were analyzed separately from those who were not.
A correlation analysis was used to evaluate whether the underlying brain electrophysiology might be the driving force of the amplitude of the P100 wave form. An increase in the EEG absolute power was significantly related (p<.001) to an increase in P100 amplitude in the occipital lobe at the O1 and O2 electrodes (O1; delta, beta, and at O2; delta, theta, alpha, and beta). However, the two were not highly correlated; the highest correlation occurred between the delta activity and the P100 amplitude, recorded at the O2 electrode; the correlation accounted for only about 20 percent of the variance in the amplitude of the P100 wave form.
Discussion
The purpose of this study was to evaluate whether EEG data predicted behaviors exhibited by a group of children and adolescents referred to a psychiatric clinic for evaluation of a wide variety of psychiatric disorders, including explosive, aggressive, and out-of-control behaviors. These individuals were referred by psychiatrists, psychologists, social workers, educators, and parents. They had been treated with little success, given various medications, and given a profusion of diagnoses.
Our study showed that individuals with a high-amplitude P100 wave form in evoked-potential evaluations and high delta band absolute power in the right frontal lobe were more likely to exhibit explosive behaviors.
It was not surprising to find that most explosive individuals had high frontal delta band absolute power (quadrants I and II in Figure 1); most experts in neurophysiology would expect such a result (Nuwer M, personal communication, 1998). Convit and associates (19) found a positive relationship between the level of violence and the amount of delta activity in psychiatric patients with violent behaviors. Fishbein and colleagues (20) reported that aggressive subjects in their study had more delta and less alpha activity in a spontaneous EEG, which they note has been observed in psychopaths and criminals.
What is new in this study is the finding that individuals with higher P100 wave form amplitudes in pattern-reversal evoked-potential studies were more likely to be explosive. Indeed, we believe that the P100, which occurs within the obligatory portion of the brain's electrophysiological response to sensory stimulation (approximately within the first 200 milliseconds after stimulus), is a biological signature and represents an individual's unique biological receptive process underlying a predisposition to process incoming stimuli in a given manner.
Although not all explosive individuals in our study had a high P100 wave form (Figure 1), the results suggest that the higher the amplitude, the more probable the explosive behaviors. Overall, these patients constitute a distinct psychiatric population in need of the unique medical treatment provided by the psychiatric community—not identification and incarceration as sociopaths by the justice system. Further studies are needed to confirm our results with children and adolescents, to investigate adult populations, to evaluate the predictability of the behavior before it is actually present, and to evaluate the relationship between the wave form and various medication regimens.
We wish to emphasize that we do not hypothesize that the high P100 amplitudes are the cause of explosive behavior. Instead, as described above, it is best to conceive of high P100 amplitudes as a marker for explosive behavior. Descending inhibitory pathways parallel the ascending sensory systems and modulate responsiveness at a very early level of processing, either at the receptors themselves or soon after, and in any event before the cortical events responsible for the generation of scalp evoked potentials (21).
As a visual stimulus moves past the retina, it proceeds down two distinct paths. The first allows it to be transported to the primary receptive area in the occipital lobes (Brodmann's area [17]). The hypothalamus also receives one-way afferent connections directly from the retina (22). This is the pathway of interest because the hypothalamus participates in autonomic or behavioral expressions of emotions as part of the survival mechanism controlled by the limbic system (23). If the intensity of the stimuli is excessive, they may cause a kindling effect as seen in seizure disorders. Andy and Velamati (24) concluded that "repeatedly recurring limbic system seizures through superkindling mechanisms can eventually render the limbic-basal ganglia-preoptico-hypothalamic aggressive system hyper-responsive to both recurring seizures and to exteroceptive stimuli with resulting aggressive behavior with or without an accompanying seizure."
These patients appear to respond positively to various combinations of anticonvulsant, antidepressant, antipsychotic, and stimulant medications. Of these, the anticonvulsants appear to deliver the most consistent benefits (5,18,25-27). Because explosive behavior appears to be a problem with emotional expression or control, defects in limbic system function have been proposed by Tancredi and Volkow (26) and by Matthews and associates (5). The positive effect of anticonvulsants may be related to the large number of gamma-aminobutyric acid (GABA) receptors located in the amygdala, a critical component of the limbic system; several of the anticonvulsants are thought to exert their effects via the GABAergic systems (17).
This study found that results for patients who were taking medication at the time of the evaluation were not significantly different from the results of those who were not. The effect of medication on visual evoked potentials, both checkerboard and flash, has been evaluated in several studies involving normal subjects and individuals with seizure disorders. The majority of studies found no effect on the amplitude of the wave forms. Although the effect of valproic acid may be nonspecific, a wide variety of anticonvulsant and sedative agents have been reported to lower the amplitude of flash evoked potentials (28). Faught and Lee (29) reported a trend toward higher amplitudes among photosensitive patients when pattern reversal was used as the visual stimulus, but the differences were not statistically significant.
No significant treatment effect was found for simple reaction time, nor was there any significant effect on either the latency or the amplitude of the visual evoked potential. Another study concluded that sodium valproate has no effect on simple reaction time or the visual evoked potential in normal subjects, although it may cause a slight increase in slow-wave sleep (30). Among patients with seizure disorders, no pronounced influence of the disorder itself on the parameters and wave form of the normal visual evoked potential using pattern reversal was demonstrated if the patients were not taking anticonvulsant drugs; the findings for patients under complete seizure control with anticonvulsants were similar (31).
The question arises as to whether the increased amplitude of the P100 wave form can be explained by the augmenting-reducing (A-R) phenomenon, also known as stimulus intensity modulation. The A-R refers to the central nervous system's modulation of responses to sensory stimuli of different intensities. In 1976 Buchsbaum (32) suggested that A-R is related to central control factors rather than peripheral mechanisms. Several authors have suggested that this central mechanism is designed to protect the cortex from overstimulation (32,33,34,35,36).
Blenner and Yingling (21) reported that their visual evoked-potential data were in agreement with those of Stenberg and colleagues (37), who found a weak augmenting pattern at both FZ and CZ but a reducing pattern at OZ. Overall they found in the visual system a slight augmentation at the vertex, but a reduction at the occiput. In our study the effect of the A-R response in our patients could not be evaluated because the stimulus intensity remained constant.
This study found a group of patients exhibiting explosive behaviors who had low-amplitude OP100 wave forms in pattern-reversal evoked-potential evaluations and low absolute-power delta (Figure 1, quadrant IV). These patients represent other subsets of individuals with explosive behaviors of different etiologies, perhaps those with temporal lobe syndrome or frontal inhibition problems. This finding is consistent with the literature indicating that several different roads can lead to explosive, aggressive behaviors. Research in our laboratory is focusing on electrophysiologically delineating these other groups. However, the importance of the amplitude of the P100 wave form in classifying and predicting explosive behaviors should not be overlooked.
The study had several limitations. First, the results are generalizable only to individuals with psychiatric problems severe enough for them to be identified by their parents, school system personnel, or health care professionals. Second, evoked potentials were recorded from scalp electrodes, and therefore the subcortical involvement identified can only be hypothesized. Third, we did not evaluate all psychophysiological response systems (for example, the electrodermal or cardiovascular) and their relationships to explosive behaviors.
Conclusions
Our findings suggest that individuals who exhibit explosive behaviors may have a predisposition for violent or explosive behavior that is an innate characteristic of their central nervous systems. The use of noninvasive visual evoked potentials and quantitative EEG data appears to allow accurate identification of this substantial subgroup of patients who have explosive disorders that appear to be biologically based. Once we identify such patients, we can direct them into the most appropriate treatment regimens.
Acknowledgment
The authors thank Marc Nuwer, M.D., Ph.D., for reviewing the manuscript.
Dr. Bars is vice-president of research and development and Dr. Heyrend is president and medical director of Behavioral Management Centers, 411 North Allumbaugh Street, Boise, Idaho 83704 (e-mail, [email protected]). Dr. Simpson is in private practice in Boise. Dr. Munger is chair of the biology department at Boise State University.
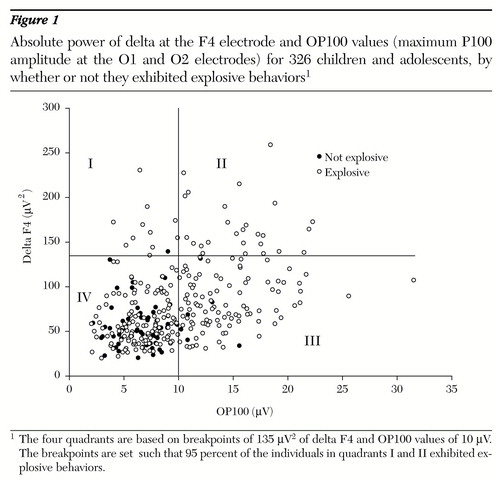
Figure 1. Absolute power of delta at the F4 electrode and OP100 values (maximum P100 amplitude at the O1 and O2 electrodes) for 326 children and adolescents, by whether or not they exhibited explosive behaviors
![]() |
Table 1. Two logistic regression models predicting behavior among 326 children and adolescents
![]() |
Table 2. Mean±SD ages and EEG values for 326 children and adolescents, by sex and by whether or not they exhibited explosive behavior
1. Stevens JR, Sachdev K, Milstein V: Behavior disorders of childhood and the electroencephalogram. Archives of Neurology 18:160-177, 1968Crossref, Medline, Google Scholar
2. Grinfeld MJ: New killing fields: will the campus shootings stop? Psychiatric Times, July 1998, pp 1-3Google Scholar
3. Cadoret RJ, Yates WR, Troughton E, et al: Genetic-environmental interaction in the genesis of aggressivity and conduct disorders. Archives of General Psychiatry 52:916-924, 1995Crossref, Medline, Google Scholar
4. Elliott FA: Neuroanatomy and neurology of aggression. Psychiatric Annals 17:385-388, 1987Crossref, Google Scholar
5. Matthews D, Williamson B, Seals J, et al: Treatment planning for violent juveniles. Presented at the annual meeting of the National Association of Private Psychiatric Hospitals, Fort Lauderdale, Fla, Jan 1993Google Scholar
6. Green JB: Association of behavior disorder with an electroencephalographic focus in children without seizures. Neurology 11:337-344, 1961Medline, Google Scholar
7. Williams D: Neural factors related to habitual aggression. Brain 92:503-520, 1969Crossref, Medline, Google Scholar
8. Fields WS, Sweet WH (eds): Neural Bases of Violence and Aggression. St Louis, Green, 1975Google Scholar
9. Mednick SA, Pollock V, Volavka JH, et al: Biology and violence, in Criminal Violence. Edited by Wolfgang ME, Weiner NA. Beverly Hills, Calif, Sage, 1982Google Scholar
10. Scarpa A, Raine A: Psychophysiology of anger and violent behavior. Psychiatric Clinics of North America 20:375-394, 1997Crossref, Medline, Google Scholar
11. Pillmann F, Rohde A, Ullrich S, et al: Violence, criminal behavior, and the EEG: significance of left hemispheric focal abnormalities. Journal of Neuropsychiatry and Clinical Neuroscience 11:454-457, 1999Crossref, Medline, Google Scholar
12. Bars DF, Heyrend FL, Simpson CD: The use of evoked potential studies in the identification of explosive adolescents. Presented at the annual meeting of the American Educational Research Association, Chicago, Apr 1991Google Scholar
13. Bars DF, Heyrend FL, Simpson CD, et al: The clinical use of computerized electroencephalography and evoked potentials in the treatment of assaultive/aggressive adolescents in a residential treatment facility. Presented at the annual meeting of the American Association of Children's Residential Centers, Nashville, Tenn, Oct 19-23, 1994Google Scholar
14. American Electroencephalographic Society: American electroencephalographic society guidelines in electroencephalography, evoked potentials, and polysomnography. Journal of Clinical Neurophysiology 11:1-147, 1994Crossref, Medline, Google Scholar
15. American Electroencephalographic Society: Evoked Potentials. Bloomfield, Conn, American Electroencephalographic Society, 1992Google Scholar
16. Lesèvre N, Rèmond A: Selected applications of a topographic approach to event-related potentials, in Topographic Mapping of Brain Electrical Activity. Edited by Duffy FH. Boston, Butterworths, 1986Google Scholar
17. Borsook D: Effect of anticonvulsants on circadian rhythms: implications for major affective disorder and primary sleep disorders, in Use of Anticonvulsants in Psychiatry. Edited by McElroy SL, Pope HG. Clifton, NJ, Oxford Health Care, 1988Google Scholar
18. Donovan J, Susser ES, Nunes EV, et al: Divalproex treatment of disruptive adolescents: a report of 10 cases. Journal of Clinical Psychiatry 58:12-15, 1997Crossref, Google Scholar
19. Convit A, Czobor P, Volavka J: Lateralized abnormality in the EEG of persistently violent psychiatric inpatients. Biological Psychiatry 30:363-370, 1991Crossref, Medline, Google Scholar
20. Fishbein DH, Herning RI, Pickworth WB, et al: EEG and brainstem auditory evoked response potentials in adult male drug abusers with self-reported histories of aggressive behavior. Biological Psychiatry 26:595-611, 1989Crossref, Medline, Google Scholar
21. Blenner JL, Yingling CD: Modality specificity of evoked potential augmenting/reducing. Electroencephalography and Clinical Neurophysiology 88:131-142, 1993Crossref, Medline, Google Scholar
22. Kupfermann I: Hypothalamus and limbic system: peptidergic neurons, homeostasis, and emotional behavior, in Principles of Neural Science, 3rd ed. Edited by Kandel ER, Schwartz J, Jessell TM. New York, Elsevier, 1991Google Scholar
23. Laddies JE: Emotion, in Handbook of Physiology: The Nervous System: Higher Cortical Functions of the Brain. Edited by Plum F, Mount Castle V. Bethesda, Md, American Physiological Society, 1987Google Scholar
24. Andy OJ, Velamati S: Limbic system seizures and aggressive behavior (superkindling effects). Pavlovian Journal of Biological Science 13:251-264, 1978Medline, Google Scholar
25. Maletzky BM: Treatable violence. Medical Times 100(10):74-79, 1972Google Scholar
26. Tancredi LR, Volkow N: Neural substrates of violent behavior: implications for law and public policy. International Journal of Law and Psychiatry 11:13-49, 1988Crossref, Medline, Google Scholar
27. Amen DG, Stubblefield M, Carmichael BA, et al: Brain SPECT findings and aggressiveness. Annals of Clinical Psychiatry 8:129-137, 1996Crossref, Medline, Google Scholar
28. Newmark ME, Penry JK: Photosensitivity and Epilepsy: A Review. New York, Raven, 1979Google Scholar
29. Faught E, Lee SI: Pattern-reversal visual evoked potentials in photosensitive epilepsy. Electroencephalography and Clinical Neurophysiology 59:125-133, 1984Crossref, Medline, Google Scholar
30. Harding GF, Alford CA, Powell TE: The effect of sodium valproate on sleep, reaction times, and visual evoked potential in normal subjects. Epilepsia 26:597-601, 1985Crossref, Medline, Google Scholar
31. Martinovic Z, Ristanovic D, Dokic-Ristanovic D, et al: Pattern-reversal visual evoked potentials recorded in children with generalized epilepsy. Clinical Electroencephalographer 21:233-243, 1990Crossref, Medline, Google Scholar
32. Buchsbaum M: Self-regulation of stimulus intensity: augmenting/reducing and the average evoked response, in Consciousness and Self-regulation. Edited by Schwartz GE, Shapiro D. New York, Plenum, 1976Google Scholar
33. Silverman I, Buchsbaum M, Henkin R: Stimulus sensitivity and stimulus intensity control. Perceptual and Motor Skills 28:71-78, 1969Crossref, Medline, Google Scholar
34. Zuckerman M, Murtaugh T, Siegel J: Sensation seeking and cortical augmenting-reducing. Psychophysiology 11:535-542, 1974Crossref, Medline, Google Scholar
35. Knorring L: The experience of pain in depressed patients: a clinical and experimental study. Neuropsychobiology 1:155-165, 1975Crossref, Medline, Google Scholar
36. Lukas J, Siegel J: Cortical mechanisms that augment or reduce evoked potentials in cats. Science 198:73-75, 1977Crossref, Medline, Google Scholar
37. Stenberg G, Rosén I, Risberg J: Personality and augmenting/reducing in visual and auditory evoked potentials. Personal and Individual Differences 9:571-579, 1988Crossref, Google Scholar